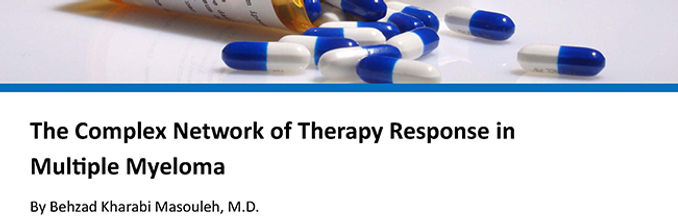
Managing Editor: Steve Mason, Ph.D.
Volume 2, Issue 2, Winter 2013
THE COMPLEX NETWORK OF THERAPY RESPONSE IN MULTIPLE MYELOMA
By Behzad Kharabi Masouleh, M.D.
The human immune system is defined by innate and adaptive responses, with the latter charged with defending the body from infectious agents. This requires the development and maturation of cells such as lymphocytes of B and T origin. B cells are known to differentiate into mature plasmaa cells, which are critical during the adaptive immune response by producing antibodies (immunoglobulins) that recognize foreign targets (antigens). This principle is used during the vaccination procedure by “training” plasma cells recognizing specific antigens, for instance against the influenza (flu) virus. Unfortunately, like every other cell type of our human body, plasma cells can undergo malignant transformation, which can lead to the plasma cell-derived malignancy multiple myeloma [1].
Although multiple myeloma treatment has steadily improved, a cure has not been identified yet. One drug class, proteasome inhibitors (PIs, e.g. bortezomib), has drastically improved response rates by prolonging overall survival of multiple myeloma patients and have therefore been established as a front-line therapy in combination treatment protocols such as VCD (bortezomib, cyclophoshamide, and dexamethasone) [2], although this usually fails to be curative [3]. Mechanistically, PIs inhibit the cellular proteasome machinery, required to salvage cells’ stress and toxic cell “waste”. Multiple myeloma cells uniquely produce high amounts of monoclonal antibodies causing extensive cell stress and making them vulnerable to PIs.
To survive stress conditions, cells (both healthy and malignant) have developed a sophisticated network of transcription factors that can activate a response termed the unfolded protein response (UPR) to enable survival. The UPR is a response that senses cellular stress through the accumulation of unfolded or misfolded proteins [4]. It consists of three major branches including the transcription factors IRE-1 and its downstream substrate XBP1 (which is spliced and thereby activated during cell stress), the enzyme protein kinase (PKR)-like endoplasmic reticulum kinase (PERK), and activating transcription factor 6 (ATF6) [5, 6]. In multiple myeloma, studies have shown the importance of the XBP1-IRE-1 pathway, where XBP1 is highly expressed. This was always suspected because of the critical role of XBP1 in plasma cell development and the increased cell stress caused by high antibody production [7].
In a recent study in Cancer Cell [8], Leung-Hagesteijn et al probed the function of XBP1, and the active XBP1s form, in resistance to multiple myeloma therapy. As a result, the authors have provided insight into the impact of two loss-of-function mutations in the XBP1 gene previously found in multiple myeloma patients that relapsed (XBP1-L167I and XBP1s-P326R) [9]. For the first time, the authors were able to provide evidence that multiple myeloma cells are not dependent on XBP1 activation, and that loss of XBP1 function has no profound effects on multiple myeloma growth. This was quite surprising and contrary to previous studies [1]. However, this was not the case for PI-induced cytotoxicity, which was quite dependent on XBP1 activation, providing a possible explanation for acquired therapy resistance in relapsed multiple myeloma patients. Additionally, the authors found evidence that these XBP1 mutations drive multiple myeloma cells towards a less mature state with decreased expression of plasma cell surface markers such as CD138 (syndecan-1). This is of particular interest since a more immature cancer cell phenotype often correlates with decreased therapy response [10]. Furthermore, the authors were able to identify specific XBP1s- progenitor cells that were enriched in therapy-resistant multiple myeloma cells, suggesting a potential reason for therapy failure.
Clinically, these findings shed new light on XBP1/XBP1s in multiple myeloma as they provide strong evidence that XBP1s activation may be dispensable for multiple myeloma growth, but is instead a potential driver of therapy resistance and subsequent failure. This is a very critical observation and has broader clinical implications in regards to novel inhibitors such as small molecule inhibitors, which directly prevent XBP1 splicing and activation by IRE-1α [11–13].
Even with these insights, there are several questions still to be addressed, and follow-up studies are required. For instance it’s not clear if these mutations render multiple myeloma cells resistant towards IRE-1 inhibitors since IRE-1 has many functions beyond splicing XBP1 [14], possibly suggesting that other XBP1-independent pathways may play an important role. Secondly, while XBP1 has been identified as a main driver of multiple myeloma growth, its broader implications in other cancer types (where XBP1 is not the only driver of the disease) is currently under investigation. Combinational therapy could be beneficial in these cases by possibly delaying the onset of XBP1 mutations. Nevertheless, these new findings are an excellent example that mutations are not always acquired due to their direct impact on a particular gene or pathway, but they may sometimes also be a consequence of secondary and even partially independent pathways.
Behzad Kharabi Masouleh, M.D., is a German physician-scientist and currently a postdoctoral fellow at the University of California, San Francisco (UCSF) studying the critical pathways underlying cell stress-mediated apoptosis in hematological malignancies.
References
1. Carrasco DR, Sukhdeo K, Protopopova M, Sinha R, Enos M, Carrasco DE, Zheng M, Mani M, Henderson J, Pinkus GS, Munshi N, Horner J, Ivanova EV, Protopopov A, Anderson KC, Tonon G, DePinho RA: The differentiation and stress response factor XBP-1 drives multiple myeloma pathogenesis. Cancer Cell 2007, 11:349–360.
2. Rajkumar SV: Multiple myeloma: 2013 update on diagnosis, risk-stratification, and management. Am. J. Hematol. 2013, 88:226–235.
3. Alexanian R, Delasalle K, Wang M, Thomas S, Weber D: Curability of multiple myeloma. Bone Marrow Res 2012, 2012:916479.
4. Hetz C: The unfolded protein response: controlling cell fate decisions under ER stress and beyond. Nat. Rev. Mol. Cell Biol. 2012, 13:89–102.
5. Scheuner D, Song B, McEwen E, Liu C, Laybutt R, Gillespie P, Saunders T, Bonner-Weir S, Kaufman RJ: Translational control is required for the unfolded protein response and in vivo glucose homeostasis. Mol. Cell 2001, 7:1165–1176.
6. Calfon M, Zeng H, Urano F, Till JH, Hubbard SR, Harding HP, Clark SG, Ron D: IRE1 couples endoplasmic reticulum load to secretory capacity by processing the XBP-1 mRNA. Nature 2002, 415:92–96.
7. Iwakoshi NN, Lee A-H, Vallabhajosyula P, Otipoby KL, Rajewsky K, Glimcher LH: Plasma cell differentiation and the unfolded protein response intersect at the transcription factor XBP-1. Nat. Immunol. 2003, 4:321–329.
8. Leung-Hagesteijn C, Erdmann N, Cheung G, Keats JJ, Stewart AK, Reece DE, Chung KC, Tiedemann RE: Xbp1s-negative tumor B cells and pre-plasmablasts mediate therapeutic proteasome inhibitor resistance in multiple myeloma. Cancer Cell 2013, 24:289–304.
9. Chapman MA, Lawrence MS, Keats JJ, Cibulskis K, Sougnez C, Schinzel AC, Harview CL, Brunet J-P, Ahmann GJ, Adli M, Anderson KC, Ardlie KG, Auclair D, Baker A, Bergsagel PL, Bernstein BE, Drier Y, Fonseca R, Gabriel SB, Hofmeister CC, Jagannath S, Jakubowiak AJ, Krishnan A, Levy J, Liefeld T, Lonial S, Mahan S, Mfuko B, Monti S, Perkins LM, Onofrio R, Pugh TJ, Rajkumar SV, Ramos AH, Siegel DS, Sivachenko A, Stewart AK, Trudel S, Vij R, Voet D, Winckler W, Zimmerman T, Carpten J, Trent J, Hahn WC, Garraway LA, Meyerson M, Lander ES, Getz G, Golub TR: Initial genome sequencing and analysis of multiple myeloma. Nature 2011, 471:467–472.
10. Kawano Y, Fujiwara S, Wada N, Izaki M, Yuki H, Okuno Y, Iyama K, Yamasaki H, Sakai A, Mitsuya H, Hata H: Multiple myeloma cells expressing low levels of CD138 have an immature phenotype and reduced sensitivity to lenalidomide. Int. J. Oncol. 2012, 41:876–884.
11. Papandreou I, Denko NC, Olson M, Van Melckebeke H, Lust S, Tam A, Solow-Cordero DE, Bouley DM, Offner F, Niwa M, Koong AC: Identification of an Ire1alpha endonuclease specific inhibitor with cytotoxic activity against human multiple myeloma. Blood 2011, 117:1311–1314.
12. Kriss CL, Pinilla-Ibarz JA, Mailloux AW, Powers JJ, Tang C-HA, Kang CW, Zanesi N, Epling-Burnette PK, Sotomayor EM, Croce CM, Del Valle JR, Hu C-CA: Overexpression of TCL1 activates the endoplasmic reticulum stress response: a novel mechanism of leukemic progression in mice. Blood 2012, 120:1027–1038.
13. Mimura N, Fulciniti M, Gorgun G, Tai Y-T, Cirstea D, Santo L, Hu Y, Fabre C, Minami J, Ohguchi H, Kiziltepe T, Ikeda H, Kawano Y, French M, Blumenthal M, Tam V, Kertesz NL, Malyankar UM, Hokenson M, Pham T, Zeng Q, Patterson JB, Richardson PG, Munshi NC, Anderson KC: Blockade of XBP1 splicing by inhibition of IRE1α is a promising therapeutic option in multiple myeloma. Blood 2012, 119:5772–5781.
14. Upton J-P, Wang L, Han D, Wang ES, Huskey NE, Lim L, Truitt M, McManus MT, Ruggero D, Goga A, Papa FR, Oakes SA: IRE1α cleaves select microRNAs during ER stress to derepress translation of proapoptotic Caspase-2. Science 2012, 338:818–822.

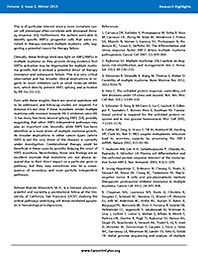
